Nanoscale modelling of natural fibres:
Carbohydrates are one of the most important classes of biomolecules, which are found in a wide range of natural materials such as wood, cotton, and paper. They play critical roles in many biological processes and are a major source of energy for many living organisms. Carbohydrates are made up of carbon, hydrogen, and oxygen atoms, and they can be classified into different types based on their structure, such as monosaccharides, disaccharides, and polysaccharides.
Cellulose is a complex polysaccharide that forms the primary structural component of the cell walls of plants. It is composed of glucose monomers that are linked together by β-1,4 glycosidic bonds. Cellulose molecules can form highly ordered structures known as microfibrils, which are the main load-bearing component of plant cell walls. Dislocated cellulose, on the other hand, refers to cellulose molecules that are in a less ordered or disordered state, often found in regions of the cell wall that undergo mechanical stress.

Hemicellulose is another class of polysaccharides that is found in plant cell walls. It is composed of a variety of different monosaccharides, including xylose, arabinose, and galactose, and it is thought to play a role in the cross-linking of cellulose microfibrils.
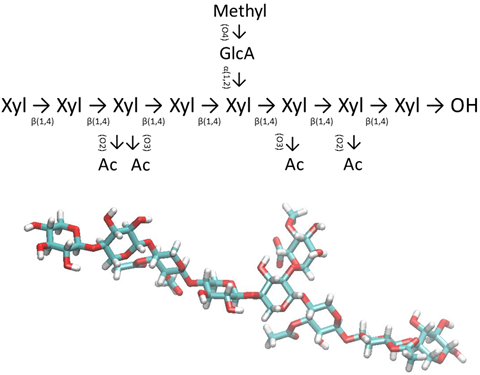
Lignin is also an important area of study. Lignin is a complex polymer that is found in the cell walls of woody plants, and it provides structural support and rigidity to the plant. Lignin is composed of phenylpropanoid units that are linked together in a three-dimensional network. Understanding the structure and behavior of lignin is important for developing new technologies for the production of biofuels and other bioproducts, as lignin is a major byproduct of the pulp and paper industry.
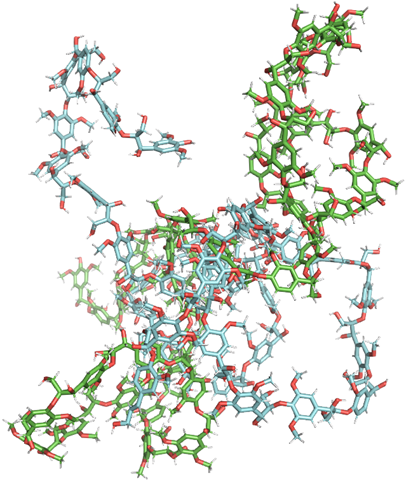
There are several computational methods that can be used for atomistic modeling of carbohydrates, such as molecular dynamics simulations and quantum mechanical calculations. These methods allow researchers to investigate the behavior of individual molecules, as well as the interactions between different molecules.
Atomistic modeling is a powerful computational technique that allows us to study the behavior of molecules at the atomic scale. In the case of carbohydrates, atomistic modeling can provide insights into their structure and dynamics, as well as their interactions with other molecules such as water. Molecular dynamics simulations, for example, can be used to study the behavior of cellulose microfibrils under different environmental conditions, such as the presence or absence of water, and can provide information about the mechanical properties of these materials. Additionally, quantum mechanical calculations can be used to study the electronic structure and reactivity of carbohydrate molecules, which can be useful for understanding their chemical and biological properties.
Overall, the atomistic modeling of carbohydrates, including cellulose, dislocated cellulose, hemicellulose, and lignin, is an important area of research with many potential applications in materials science, biotechnology, and bioenergy. At the Department of Materials Engineering, KU Leuven, we have maintained focus on modelling studies of carbohydrates for more than 5 years now. Our studies aim to provide atomistic insights on the mechanical, rheological, and thermodynamical aspects of the plant cell walls.
Tensile behaviour of cellulose and dislocated cellulose:
Atomistic modelling of cellulose has been extensively studied using molecular dynamics simulations. In a recent study, researchers modeled Iβ crystalline cellulose and developed a model that includes dislocations between the crystal regions.1 The model including dislocations showed a tensile modulus of 109 GPa, which is 25% lower than that of the fully crystalline model (146 GPa). The change in dihedral angle preferences was analyzed, and its effect on hydrogen bonding pattern was assessed. The presence of hydrogen bonds was found to contribute to the elastic properties of cellulose nano-fibrils. The effect of water on the elastic modulus of fibrils was also investigated. Moreover, the researchers provided an illustration of how the tensile behavior of fibrils is controlled by a synergy between the geometry changes occurring at the glycosidic linkage, reflected by specific torsional and glycosidic angles. These findings could be useful in further modeling of cellulosic fibrils at the atomistic and coarse-grained scales.

Another study focused on the development of a micromechanical model, the inverse rule of mixtures, to predict the elastic modulus of multi-component composites, such as cellulose nano-fibrils, when load is perpendicular to the stratified structure, by implementing molecular dynamics simulations.2 The inverse rule of mixtures was found to predict the tensile modulus of the modeled cellulose nano-fibrils in the fibril direction. These findings could be generalized to compute the mechanical properties of multi-phase nanocomposites, in case the constituents are loaded in series, as well as to approximate the degree of crystallinity of single cellulose nano-fibrils.
In summary, these two studies shed light on the mechanical properties of cellulose nano-fibrils. The first study demonstrated the impact of dislocations on the elastic modulus of cellulose nano-fibrils. The presence of dislocations in the fibril structure resulted in a lower tensile modulus, which could be attributed to a change in the dihedral angle preferences and the hydrogen bonding pattern. Additionally, the study showed how specific torsional and glycosidic angles affect the tensile behavior of fibrils. The second study introduced a micromechanical model that can predict the elastic modulus of multi-component composites, including cellulose nano-fibrils, in the fibril direction. This model could be used to approximate the degree of crystallinity of single cellulose nano-fibrils.
These findings could be relevant in various fields, such as material science, nanotechnology, and biotechnology, where cellulose is used as a key component in various applications. The knowledge gained from these studies could inform the design of novel cellulose-based materials with tailored mechanical properties.
The above two studies were performed by non-reactive molecular dynamics. In particular, non-reactive simulations are able to study the dynamics in a more time-efficient manner, however, studies cannot consider formation or breakage of the bonds, and hence, chemical reactions cannot be considered in non-reactive molecular dynamics. To this end, our researchers have also focused on the reactive side of cellulosic fibrils and have performed extensive reactive atomistic studies.
Ultimate properties of cellulose nanocrystals and failure mechanisms:
In another study, our researchers aimed to investigate the mechanical properties of cellulose nanocrystals (CNCs) using reactive molecular dynamics simulations.3 While CNCs offer excellent mechanical properties, measuring their strength through experiments at the nanoscale is challenging. Therefore, our researchers developed a model of Iβ crystalline cellulose and inspected the structural changes and hydrogen-bonding characteristics of CNCs during a tensile test.
We took into account the fibril twist in the model and analyzed the failure mechanism of CNCs down to the scale of individual bonds. We found that the C4–O4 glycosidic bond was responsible for the failure of CNCs. The effect of strain rate on ultimate properties was also analyzed, and a nonlinear model was used to predict the ultimate strength of 9.2 GPa and ultimate strain of 8.5% at a 1 s-1 strain rate.
The findings of this study shed light on the applications of cellulose in nanocomposites and further modeling of cellulose nanofibers. The knowledge gained from this study can be useful for designing and developing nanocomposites with improved mechanical properties. Additionally, the results of this study may aid in the development of new models for predicting the mechanical properties of CNCs under various conditions. Our research findings may pave the way for the development of new materials with improved mechanical properties, which can have significant implications for a wide range of applications.
Size distribution of dislocations in cellulose microfibrils:
Another area of research in the field of atomistic modeling of carbohydrates is the arrangement of cellulose molecules in natural environments on the nanoscale. One question that has been debated is the length of dislocated cellulose segments in cellulose microfibrils (CMF) due to longitudinal disorder. Molecular dynamics simulations have been used by our researchers to investigate the phenomenon of pseudo-recrystallization of dislocated cellulose regions after cleavage of CMFs.4 Based on these simulations, the results propose that 3-4 glucose residues bordering each side of a cellulose nanocrystal are reorganizing into a quasi-crystalline state, which is supported by recent analytical investigations reporting an increase in crystallinity after acid vapor hydrolysis of CMFs. Below is an example of the computational X-Ray diffraction patterns of the crystalline cellulose, dislocated cellulose, and the reorganized state after cleavage.
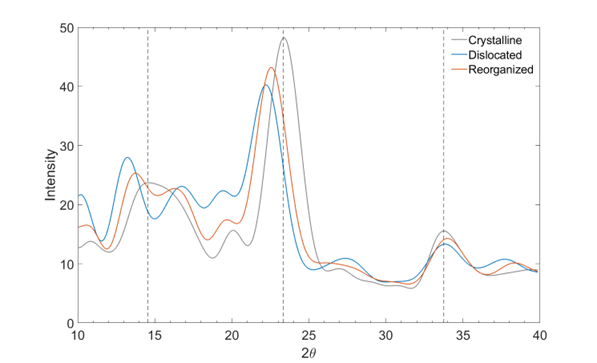
We combined our molecular dynamics simulation results with analytical data from the literature to estimate the length of the dislocated cellulose segments in CMFs. For the investigated sources of biomass (cotton and ramie), the dislocation lengths are proposed to be between 3.1-5.8 nm, equivalent to 6-11 glucose residues in the cellulose crystallites. These findings provide insights into the arrangement of cellulose molecules in natural environments and can be used to inform further modeling of cellulosic fibrils at the atomistic and coarse-grained scales.
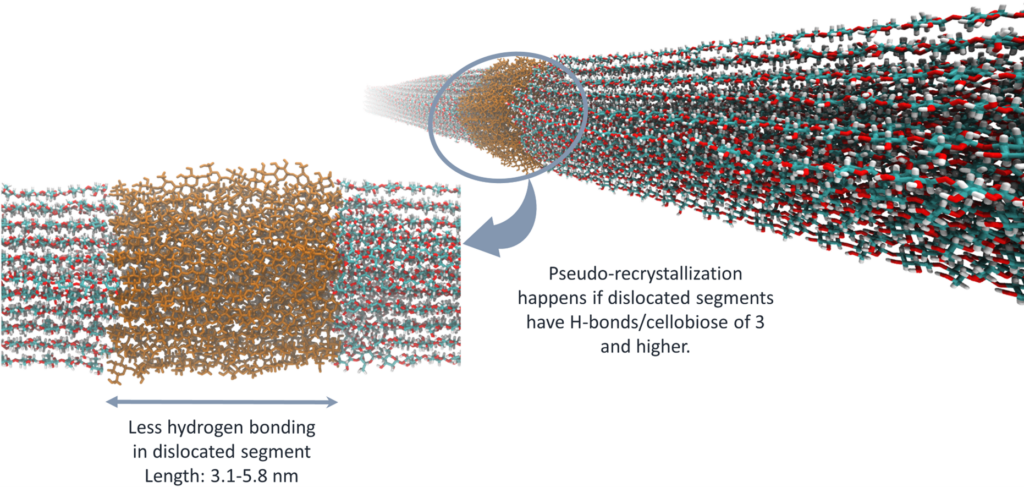
Overall, atomistic modeling of carbohydrates, particularly cellulose, is a complex area of research that involves investigating various properties and phenomena. The development of models that include dislocations in between crystal regions has shown a lower tensile modulus compared to fully crystalline models. The effect of dihedral angle preferences on the hydrogen bonding pattern and the contribution of hydrogen bonds to the elastic properties of cellulose nano-fibrils have been explored. The impact of water on the elastic modulus of fibrils has also been investigated. Furthermore, the inverse rule of mixtures has been used to predict the elastic modulus of multi-component composites such as cellulose nano-fibrils. Finally, the length of dislocated cellulose segments in cellulose microfibrils has been estimated through molecular dynamics simulations, providing insights into the arrangement of cellulose molecules in natural environments.
Interaction of hemicellulose with cellulose in the plant cell wall:
To step further and inspect the interactions between the consisting elements in the plant cell wall, we investigated the interactions between different hemicellulose models and their respective binding strength to cellulose nanocrystals using molecular dynamics simulations.5
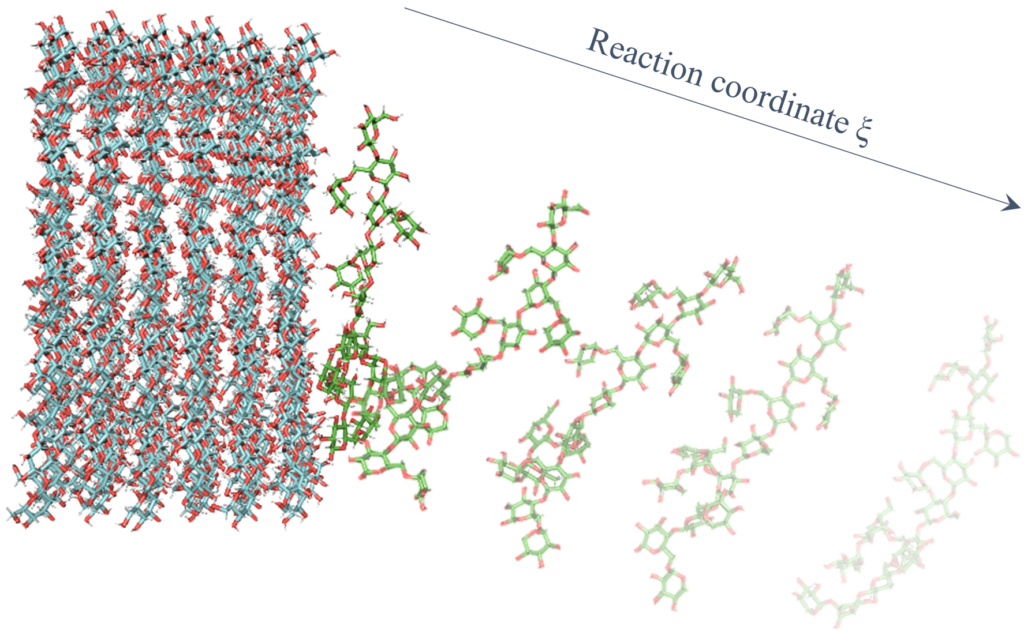
The binding strength of five different hemicellulose models, including Galactoglucomannan, O-Acetyl-Galactoglucomannan, Fuco-Galacto-Xyloglucan, 4-O-Methylglucuronoxylan, and 4-O-Methylglucuronoarabinoxylan, to cellulose nanocrystals was analyzed, and it was found that glucuronoarabinoxylan showed the highest free energy of binding. The presence of water molecules had a weakening effect on the interactions between hemicellulose and cellulose, as water molecules take up the available hydroxyl groups on the surface of the cellulose for hydrogen bonding.
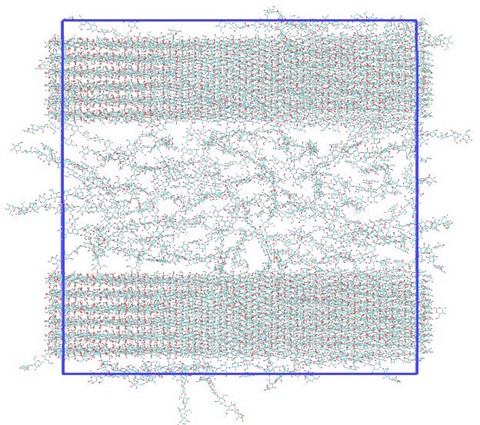
In previous studies discussed earlier, the elastic properties of cellulose nano-fibrils, including their tensile modulus and effect of water on the elastic modulus, were investigated using molecular dynamics simulations. The effects of dislocations in cellulose microfibrils and their length in CMFs were also studied using molecular dynamics simulations. By combining the findings of these studies with the current study on hemicellulose interactions, a better understanding of the structure and mechanical properties of plant cell walls can be gained, which is useful for applications such as extractions, purification, and operation of biorefineries.
Overall, the results of this study provide insights into the variability of the structures of different plant sources and their interactions with cellulose nanocrystals, which can be useful for optimizing the extraction and processing of plant materials in various industries. The combination of these studies on atomistic modeling of carbohydrates, specifically cellulose, dislocated cellulose, hemicellulose, and lignin, in the presence and absence of water, can pave the way for the development of more efficient and sustainable methods for the production of biofuels, paper products, and other materials derived from plant sources.
References:
[1] Khodayari, A., Van Vuure, A. W., Hirn, U., & Seveno, D. (2020). Tensile behaviour of dislocated/crystalline cellulose fibrils at the nano scale. Carbohydrate Polymers, 235(October 2019), 115946. https://doi.org/10.1016/j.carbpol.2020.115946
[2] Khodayari, A., Hirn, U., Van Vuure, A., & Seveno, D. (2020). Inverse rule of mixtures at the nanoscale: prediction of elastic properties of cellulose nanofibrils. Composites Part A: Applied Science and Manufacturing, 138(July), 106046. https://doi.org/10.1016/j.compositesa.2020.106046
[3] Gupta, A., Khodayari, A., Van Duin, A. C. T., Hirn, U., Van Vuure, A. W., & Seveno, D. (2022). Cellulose Nanocrystals: Tensile Strength and Failure Mechanisms Revealed Using Reactive Molecular Dynamics. Biomacromolecules, 23(6), 2243–2254. https://doi.org/10.1021/acs.biomac.1c01110
[4] Khodayari, A., Hirn, U., Spirk, S., Van Vuure, A. W., & Seveno, D. (2021). Recrystallization and size distribution of dislocated segments in cellulose microfibrils—a molecular dynamics perspective. Cellulose, 28(10), 6007–6022. https://doi.org/10.1007/s10570-021-03906-7
[5] Khodayari, A., Thielemans, W., Hirn, U., Vuure, A. W. Van, & Seveno, D. (2021). Cellulose-hemicellulose interactions – A nanoscale view. Carbohydrate Polymers, 270(15 October), 118364. https://doi.org/10.1016/j.carbpol.2021.118364